Research
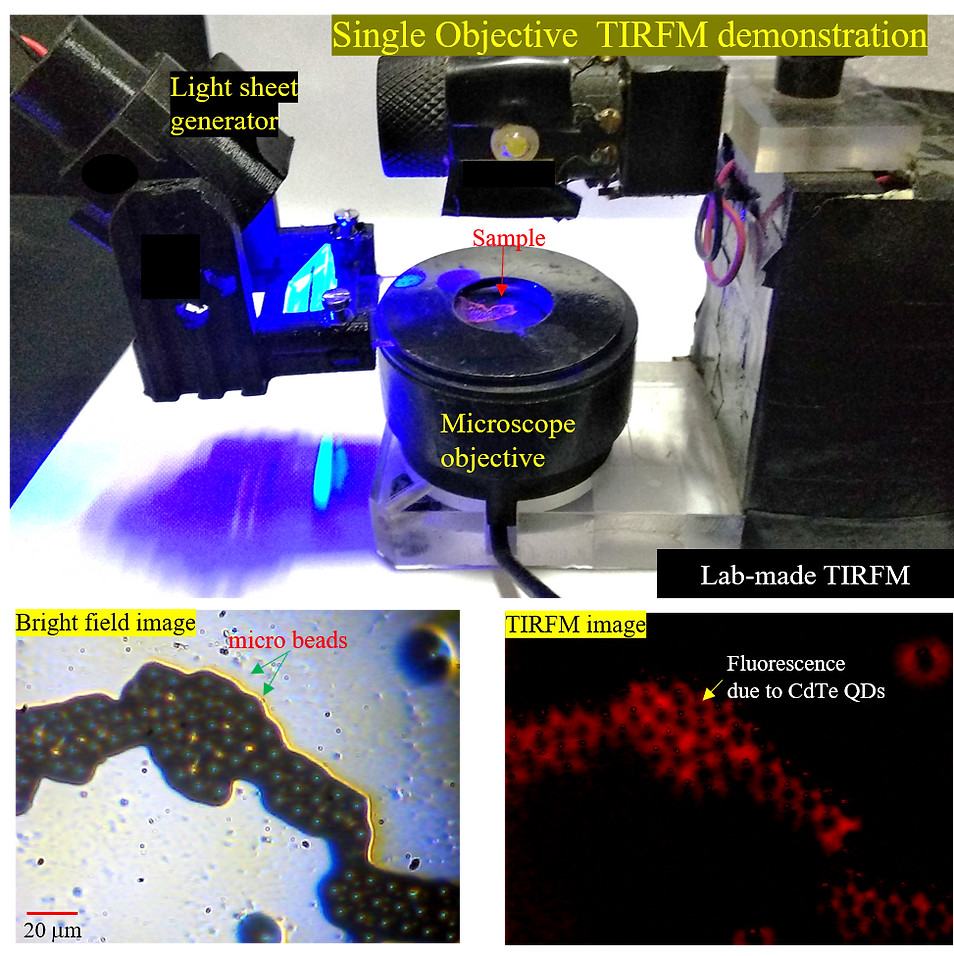
Soft Aspheric Lens and Portable Optical Microscope
Optical lenses are used in a diverse spectrum of scientific and engineering applications starting from optical imaging devices such as telescope, microscope, and optical camera to data storage devices such as CD drive or 3D optical data storage etc. Regardless of these many different applications, a spherical optical lens is associated with a common problem known as spherical aberration, which causes imperfection in captured image. This problem has been sought to be resolved by combining many different optical components such as bi-convex, meniscus, and bi-concave lenses aligned axially together to make a compound lens that produces aberration free image. However, construction of a compound involves sophisticated instrumentation, requires sufficient infrastructure and most importantly they are bulky. In addition, a different approach for the solution has been adopted by modulating the profile of the lens such that it diminishes the spherical aberration by converging paraxial and marginal rays at a single point on the optical axis. Such types of lenses are known as aspherical lens. However, conventionally curving the profile of a brittle solid other than spherical shape is challenging and requires precision machines, sophisticated control algorithms, accurate control of temperature, humidity, surrounding vibration and importantly highly skilled workmanship. To overcome these shortcomings, we have made use number of self-assembled phenomena occurring in soft polymeric materials to produce exotic yet highly controlled aspherical optical lenses. These soft lenses have the advantage that unlike brittle materials, these are resistant to surface defects like roughness.
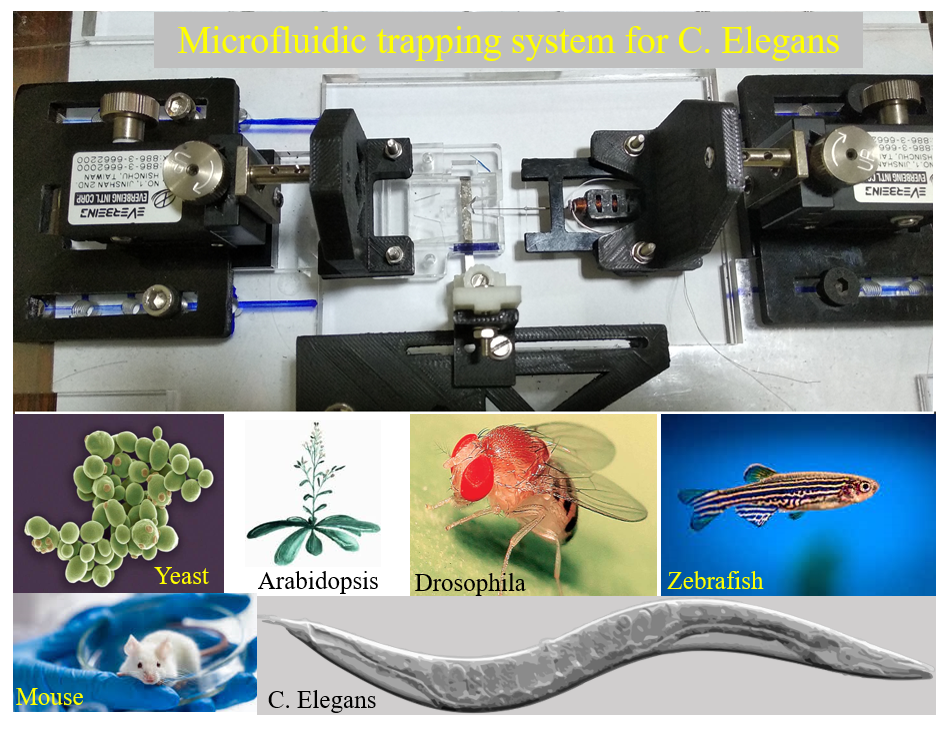
Bio-MEMS
In developmental biology, the researcher uses model organisms such as Yeast, Drosophila, Arabidopsis, Mouse, Zebrafish, etc. Similarly, C. elegans is also such type of model organism, one type of earthworm. Various types of studies were been performed on this model organism. However, studies like the mechanoresponsive behavior of the C elegans need to be done on a stationary C. elegan, but one of the characteristics of C. Elegans is that it continuously crawls on a surface. Also, we need an actuator capable of exerting micro Newton force to the C. Elegans. Therefore, we have developed a micro-fluidic trapping system for C. elegans for the study of the mechanoresponsive behavior of C. Elegans. A three-dimensional soft lithographic layer by layer process has been utilized to make the trapping system. The system is made in such a way that a channel can be created to insert the actuator probe to induce the mechanical stimuli to the subject. A compliant based electromagnetic actuator in the force range of 1-40uN is developed for the stimulation of the C. Elegans.
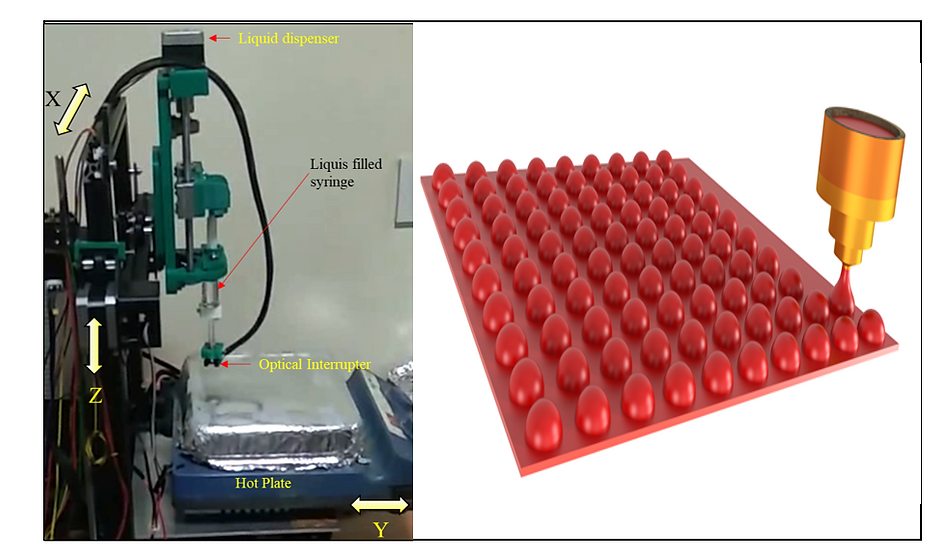
Liquid polymer 3D printing
We have developed a technique to print liquid polymeric materials using rapid arresting of the spreading of thermally cross-linkable polymers. For various purposes including making of soft electronic skin.

Optofluidics sensors
We are working on various on-chip optofluidic sensors. One such example includes our recently published work on surface deformable liquid Dove prism (LDP) based low-fluid flow pressure monitoring devices. The unique design of the device in combination with liquid and soft solid enabled by the total internal reflection of light makes the sensor highly sensitive and compatible with the integration of a microfluidic and/or Lab-on-a-chip device. A layer-by-layer soft lithographic (LSL) and 3D printing technique is exploited to make the device. We have used Polydimethylsiloxane (PDMS) as the layer material and two varieties of liquids (i) immersion oil (IO) and (ii) di-iodomethane (DI) as refracting medium to construct the LDP sensor. Optical ray tracing simulation is performed to optimize the sensor. The pressure sensor shows sensitivity as high as ±28.5 per 50 pressure with an error ± 2.5 and repeatability of ~99.56% at full scale. We have shown the applicability of the sensor by capturing and analyzing respiratory pressure signals of some human subjects under numerous conditions.
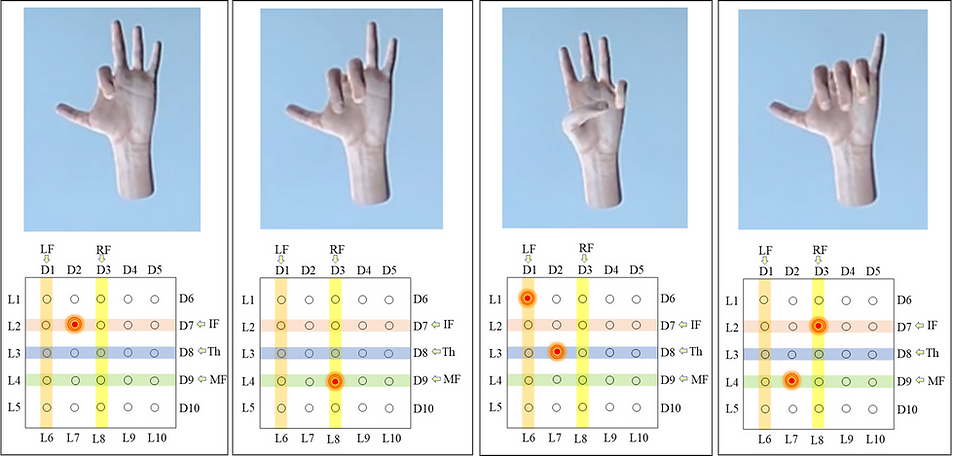
Soft Electronic Skin
Soft electronic skin (soft-e-skin) capable of sensing touch and pressure similar to soft human skin is important in many applications including robotics, healthcare, augmented reality etc. However, most of the research effort on soft-e-skin confined to lab-scale demonstration. Several hurdles still remain challenging such as highly complex and expensive fabrication process, shows instability at long-term and difficulty in producing large area and mass production etc. Therefore, we are working on a robust 3D printable large area electronic skin made of a soft and resilient polymer with extreme sensitivity (up to ) to touch and load. The soft-e-skin shows excellent long-term stability i.e. it shows consistent performance up to almost a year. Also, we describe a fabrication process capable of producing large areas, large numbers yet cost-effective. The soft-e-skin consists of two layers of soft membrane, uniquely designed layers that work exclusively as a soft optical waveguide and the other layer consists of an array of soft hemispherical structures work as passive sensing nodes. The use of a hemispherical structure gives the liberty of stretching to the soft-e-skin without considering the disjoints among the sensing nodes. We have shown the functioning of the soft-e-skin in various means.
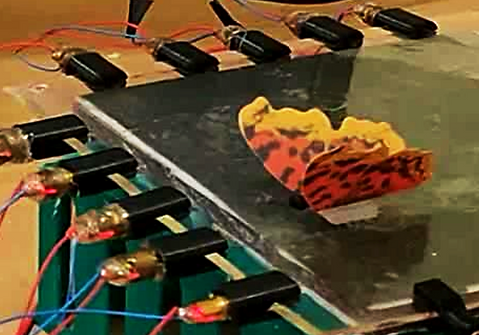
Soft Electronic Skin
Publications
Abhijit Chandra Roy*, Rudresh S. M, and V. Venkataraman, On-chip optofluidic low-pressure monitoring device, Journal of Biophotonics (2021), 14, 1-13. (Cover article, Corresponding author, IF- 3.06)
Abhijit Chandra Roy*, Navin Kumar, Shreyas Bangalore Subramanya, Ananya Gupta, Aloke Kumar, Aveek Bid*, and Venkatakrishnan Venkataraman, Large-Area 3D Printable Soft Electronic Skin for Biomedical Applications, ACS Biomaterial science and Engineering, (ASAP article) (https://pubs.acs.org/action/showCitFormats?doi=10.1021/acsbiomaterials.2c00241&ref=pdf) (Cover page article, Corresponding author, IF- 5.4)
Abhijit Chandra Roy, and A. Ghatak. Design of an adaptable optofluidic aspherical lens by using the elastocapillary effect. Adv. Optical Mater. (2014), 2, 874–878. (Cover article, IF~ 8.2)
Abhijit Chandra Roy, M. Yadav, E. P. Arul, A. Khanna. and A. Ghatak. Generation of aspherical optical lenses via arrested spreading and pinching of a cross-linkable liquid. Langmuir (2016), 32, 5356−5364 (IF~3.6).
Abhijit Chandra Roy, M. Yadav, A. Khanna and A. Ghatak. Bi-convex aspheric optical lenses. Appl. Phy. Lett. (2017), 110, 103701. (News, IF ~ 3.6)
Abhijit Chandra Roy, V.S. Nisha C. Dhand, Md. A. Ali, B.D. Malhotra. Molecularly imprinted polyaniline-polyvinyl sulphonic acid composite-based sensor for para-nitrophenol detection. Analytica Chimica Acta. (2013), 777, 63– 71(IF~6).
D. Hore, A. Majumder, S. Mondal, Abhijit Chandra Roy, and A. Ghatak. How to make a cylinder roll uphill. Soft Matter. (2012), 8, 5038. (News, IF 3.4)
Abhijit Chandra Roy, and D. Mohanta, Structural and ferroelectric properties of solid-state derived carbonate-free barium titanate (BaTiO3) nanoscale particles. Scripta Materialia. (2009), 61, 891–894 (IF~5).
Abhijit Chandra Roy, & D. Mohanta, Optimum Mn-doping, effective tetragonality, and correlated luminescence characteristics of PbTiO3 nanoparticles. Philosophical Magazine Letters. (2011), 916, 423–431(IF~1.3).
Abhijit Chandra Roy*, Amruta Vasudevan, Siddharth Khare, Sandhya Koushika and V. Venkataraman, A complete kit for neural signaling study for C. elegans: A trapping chamber and an electromagnetic force actuator. Sensors and Actuators B: Chemicals (preparation ongoing)
Shreyas B.S, Shankar Srinidhi, Sumana E. N, and Abhijit Chandra Roy*, Ultra-flexible optical sensor capable of measure strain, load, and torsion. Advanced Materials (preparation ongoing)
Conference Publications
Abhijit Chandra Roy, and D. Mohanta. A comprehensive study of perovskite barium titanate ferroelectric nanoparticles and prospective application in life sciences, 96-Indian Science Congress held in at shillong-2009, India
Abhijit Chandra Roy, and D. Mohanta. Studies on the technologically important carbonate free ferroelectric nanostructure and their biosensing applications. -2009. International Conference on Materials for Advanced Technologies (ICMAT). Singapore, 2009.
Abhijit Chandra Roy, and D. Mohanta. Cost effective synthesis and characterization of barium titanate (batio3) and lead titanate (PbTiO3) nanoparticles. International Conference on Optics and Photonics CSIO, Chandigarh, India, 30 Oct.-1 Nov. 2009.
Abhijit Chandra Roy, and A. Ghatak. Design of soft optofluidics devices using solid-fluid interfacial and bulk properties. The Advanced Materials World Congress (AMWC), 23-26 August, Stockholm, Sweden-2015.
Abhijit Chandra Roy, Navin Kumar, B S Shreyas, Ananya Gupta, Aloke Kumar, and V. Venkataraman, Optical soft artificial skin, Frontiers in Optics 2021, Washington, DC United States, 1–4 November 2021.
Patent Filed
1.Abhijit Chandra Roy, Aveek Bid, Process of making aspherical lenses and mirrors, MPS-PHY-2023-004, 2022
2.Abhijit Chandra Roy, Aveek Bid, System to monitor and assist finger movement. Application No. 202241045417, 2022.
3. Abhijit Chandra Roy, Aveek Bid, Wearable sensing devices. Application No. 202241045416, 2022.
4. Abhijit Chandra Roy, Navin Kumar, Aloke Kumar, Apparatus for detecting a touch position Application No. 202041056193, 2020.
5. Abhijit Chandra Roy, Liquid dove-prism based optofluidic low-pressure sensor. Application No. 202041039038, 2019.
6. Santosh Pramanik, Kaniska Biswas, Abhijit Chandra Roy, Method for Fabricating Microstructures, 201711042059, 23.11.2017, Grant no. 334378 (Grant date: 11.03.2020).
7. Animangsu Ghatak, Abhijit Chandra Roy, Mr. Ravindra Vishwakarma Microscope System & Method, 201711034629, 29.09.2017.
8. Animangsu Ghatak, Abhijit Chandra Roy, Mr. Ravindra Vishwakarma, A Smartphone-Based Microscope, 201711028373, 10.08.2017.
9. Animangsu Ghatak, Abhijit Chandra Roy, Mr. Mridul Yadav, A Method of Fabrication of Biconvex Optical Lens and Optical Lens Thereof, 201611043743, 21.12.2016.
10. Animangsu Ghatak, Abhijit Chandra Roy, Methods for Fabricating Optical Lenses EP– 14866113, 17.11.2014.
11. Animangsu Ghatak, Abhijit Chandra Roy, Methods for Fabricating Optical Lenses US- 15/100,328, 17.11.2014.
12. Anumangsu Ghatak, Abhijit Chandra Roy, and Mr. Edward Peter Arul, An Optical Lens, Lens Filter and Preparation Thereof, 1964/DEL/2014, 12.07.2014.
13. Animangsu Ghatak, Abhijit Chandra Roy, Methods for Fabricating Optical Lenses, 3473/DEL/2013, 29.11.2013.
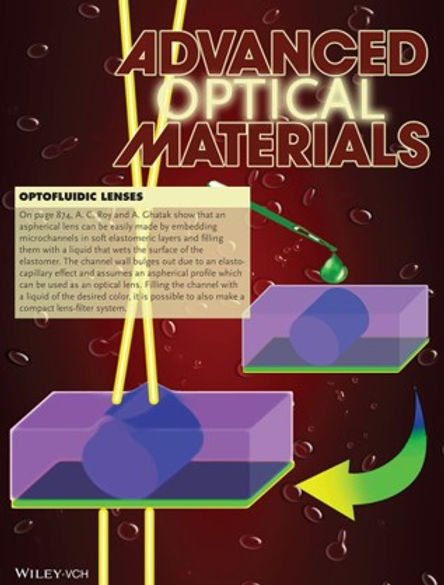
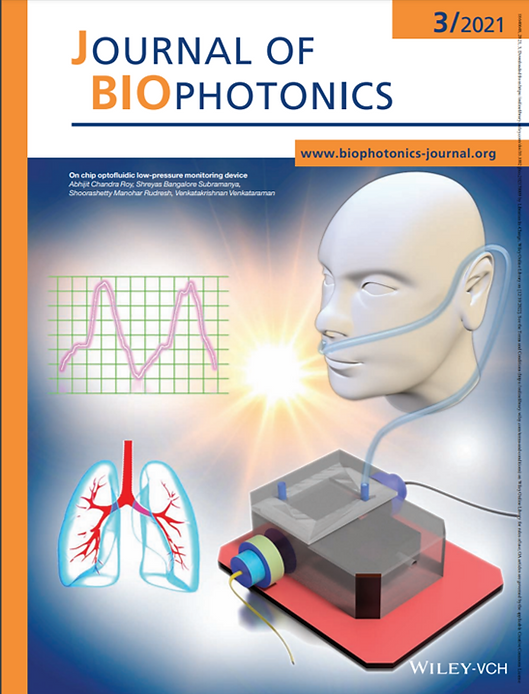
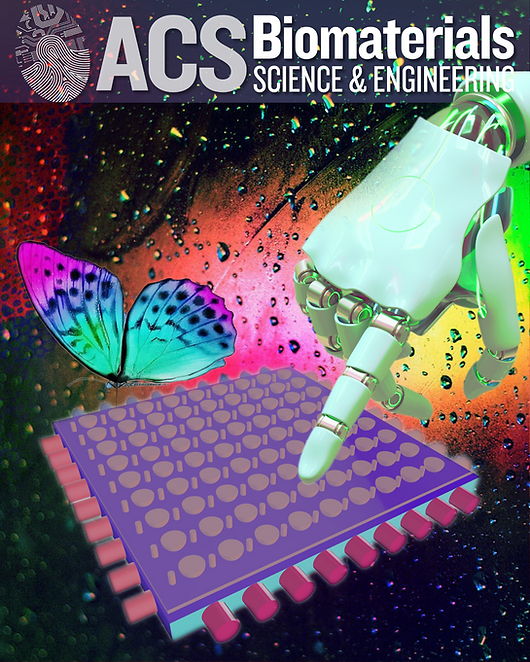
Upcoming Research
Self-powered Soft-e-skin with the capability of sensing temperature, humedity and light intensity
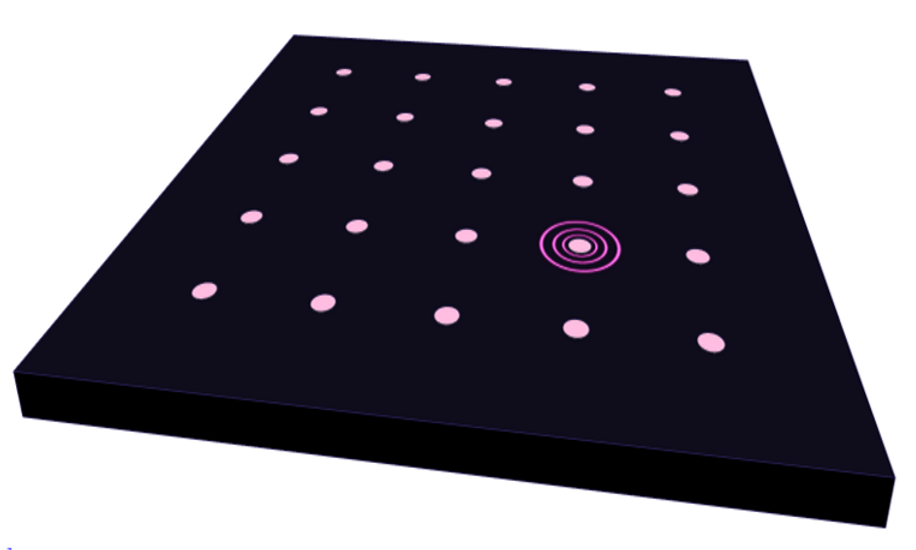
We have already developed soft, stretchable, and electronic skin based on specially designed optical waveguide and soft structures capable of measuring touch and load. However, in many instances, knowledge of local temperature and humidity may be very essential for applications such as robotics, the internet of things, and healthcare. Therefore, we are working on developing soft electronic skin capable of detecting touch, load, temperature, humidity, and light intensity using a biomimetic route.
Artificial Intelligence based portable microplate reader for Point-of-Care applications

The project is all about developing a handheld, cost-effective, and user-friendly microplate reader using specialized design enabled by 3D printing and Artificial Intelligence (AI). In the recently emerging field of computer science namely AI and additive manufacturing 3D printing techniques, some of the conventional processes can be done more efficiently and cost-effectively by using them individually or in a combined way. A few years back some works appeared to be impossible, today it becomes possible due to the emergence of AI and 3D printing. AI helps in understanding patterns and correlate them to some meaningful relation despite their not so well scientific law to express them. On the other hand, 3D printing empowers us in making complex organic shapes that are otherwise very difficult and expensive to make using a conventional process. Therefore, in this project, our focus is to take advantage of these two emerging technologies to make a compact, handheld, and cost-effective microplate reader without compromising the high quality of the device. Although microplate reader has applications in many fields such as the Food industry, Water quality, Medicine, Bacteriology, Virology, etc, however, Enzyme-linked Immunosorbent Assay (ELISA) has one of the important applications in various disease diagnostics and drug development. Considering the disease diagnostic application of the microplate reader, there is a consistent demand for cost-effective diagnostic devices in resource-limited areas around the globe. Also, portability and compactness of the device are additional important aspects of the demand for medical diagnostic in resource-constrained remote locations. However, commercially available microplate readers used for ELISA are bulky and highly expensive, not suitable for field studies in remote locations with resource-limited settings. Although, some alternative technologies for ELISA are commercially available in the market such as lateral flow devices (LFA) point-of-care (POC) immunoassays, however, LAF has significant shortcomings compared to enzyme-linked immunosorbent assay-based tests. For example, compared to ELISA, lateral flow assays (LFAs) have much lesser sensitivity and it’s due to the formation of limited interactions between antigen and antibody in the case of LAF. Besides, LFAs provide a qualitative analyte assessment of the test which restricts the usefulness for vaccine formulations and deadly disease diagnostics. Lastly, LFA is usually designed for single use only resulting in hindrance for large populations screening due to high expense and time consumption. Therefore, keeping these shortcomings LFA and advantages of ELISA, we have started developing a 3D printing and AI-based compact handheld microplate reader suitable for various applications including disease diagnostic using Enzyme-linked Immunosorbent Assay (ELISA). Again, according to the market research agency the Technology Network#, there is a demand for the image capturing facility for the microplates to correlate the result with the images of the wells of the microplate to increase the reliability of the result. Again, reliability is one of the important factors that determine the market of a device.
Portable Total internal reflection fluorescence microscopy (TIRF)

Total internal reflection fluorescence microscopy (TIRF) is an essential imaging tool that facilitates to image a very thin layer (~< 200 nm depth) of near-surface and interfacial processes at the sample and glass slide interface without background noise. TIRF microscope finds usefulness in numerous scientific studies such as molecular genetics [1] DNA biophysics [2], biosensor design [3], and basic separation theory [4], etc. Moreover, the TIRF microscope is also an essential tool to study various molecular activity at the cell plasma membrane which includes kinetic rates of binding proteins to cell surface receptors [5], membrane trafficking [6], cytoskeleton remodeling [7], cell adhesion [8], cargo transport by molecular motor [9], single-molecule imaging [10] and nanoparticle-membrane interaction events for targeted drug delivery [11] etc. Despite these many important applications of the microscope, the use of this microscope sometimes is limited by its expensiveness and some inherent drawbacks.
The conventional TIRF microscope uses either prism or high numerical aperture objective lens to generate evanescent waves at the interface. In a prism-based TIRF microscope, the evanescent field is generated at the coverslip/buffer interface through a prism which directs the laser light towards the coverslip. Although prism-based TIRF generates a thin and clean evanescent field without undesirable disturbance due to reflected light, its not free from remarkable shortcomings such as it is bulky, complex to set up, and usually expensive. Moreover, the prism-based TIRF microscope is associated with direct coupling of the sample with the prism which limits the sample movement and often requires unconventional sample mounting systems. On the other hand, In an objective-based TIRF microscope, an objective lens with a high numerical aperture (N.A) ( >1.4) is used for the purpose of both delivery of light as well as the collection of fluorescence light from the sample. Even though a high objective-based TIRF microscope is easy to use, however, typically too expensive and generates background noise due to specular reflection from the incident light as the same objective is used to deliver and collect the light. In addition, a conventional TIRF microscope uses laser light to produce enough intensity of evanescence wave to excite the fluorescent molecule. This typically expensive laser source adds an extra expense to the conventional TIRF microscope.
Therefore, in recent times, researchers have noticed those limitations of the conventional TIRF microscope and come up with some innovative idea of introducing low-cost LED light source and waveguide-based evanescence field generation to resolve the problems confronted by conventional TIRF microscope. However, despite the introduction of LED and waveguide-based smart configuration of these recent reports, LED light may not produce enough evanescence field intensity compared to a laser light source, which remains insufficient for a single objective TIRF microscope to collect maximum possible photons emitted by the fluorescence molecule. Therefore, LED as a light source may not give the expected result when a single objective TIRF microscope is used to collect the fluorescence light.
Therefore, we have come up with a novel configuration to overcome the possibility of insufficient evanescence field strength due to the use of a LED light and enhance the light collection capability of the TIRF microscope from the fluorescence molecule. The problem is overcome by introducing dual aspherical objective lenses facing each other for collecting light from both sides of the sample and, also proposed a smart design of waveguide to sufficiently enhance the evanescence field due to a LED light source. The dual objective lens configuration can not only give more information about the specimen but also each objective lens can focus on a different 2D plane to capture movements of the interfacial events at different focal planes. The proposed waveguide is modified by introducing a reflecting coating on the oppositely facing wall of the waveguide to reduce the loss of light coming out from the waveguide. Moreover, we have proposed to use custom-made cost-effective aspherical objective lenses and other necessary optical components to make the device cost-effective and compact (Earlier work )
Development of 3D bioprinter
Millions of people die Globally due to organ failure and lack of a suitable organ donor. Therefore, many researchers are engaged in recently emerging 3D printing techniques to print various organs to save a life. Similar to conventional 3D printing, 3D Bioprinting involves layer-by-layer dispensing of biological cells on a biocompatible scaffold platform to make a three-dimensional structure similar to human organs. However, one of the drawbacks of such 3D bioprinter is the incompetency of providing blood vessels, or urine circulating tubes, etc. The lack of these makes the 3D printed organ incapable of circulating essential nutrients and oxygen deep within their interiors. Therefore, in this project, we will be working on introducing soft biomimetic micro-channels inside the organs during the layer-by-layer 3D printing process.
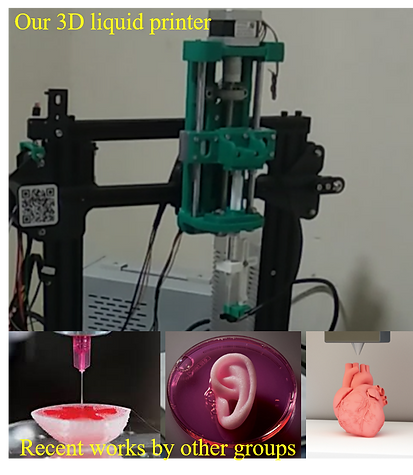
Development of micropump suitable for microfluidic application
CONTACT
Dr. Abhijit Chandra Roy
Research Manager
Q-Line Biotech Private Limited
Technopark IIT Kanpur
Ex-DST INSPIRE Faculty
Department of Physics
IISc Bangalore, India
Email: abhijit.ch.roy1@gmail.com
abhijit.roy@qlinebiotech.com
https://www.qlinebiotech.com/
https://abhiphn09.wixsite.com/softbioelectrolab